Precious laboratory samples including DNA and RNA, which are used for genetic engineering, are stored in liquid nitrogen freezers like this one to keep them stable.
A MOUSE MODEL TO EVALUATE LIVE ATTENUATED VACCINE CANDIDATES
- Jan 1, 2016
- Download Retrospective
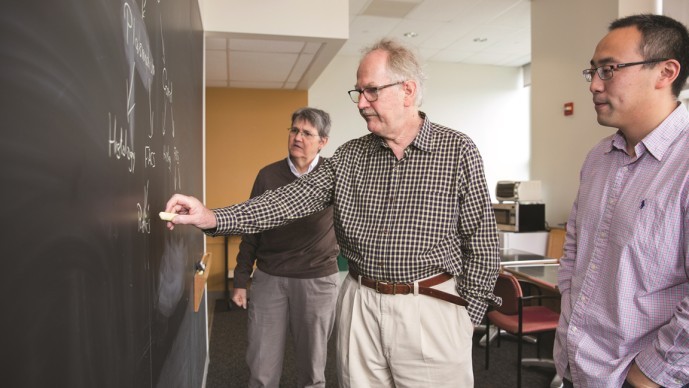
Richard Flavell discusses an experimental strategy with colleagues for using their humanized mice to study malaria parasites and test candidate vaccines.
Richard Flavell, Yale University School of Medicine, United States
Professor Richard Flavell was uncharacteristically worried. It was July 2009 and Flavell, a renowned immunologist at Yale University, had just received word that his ambitious project to transform vaccine research was going to be shut down years before it could be completed.
In 2005, Flavell had received a five-year, $17 million grant from the Bill & Melinda Gates Foundation's new Grand Challenges in Global Health (GCGH) program. It was the single largest research funding the veteran scientist had ever received, one of 44 unusually generous grants worth a total of about $450 million awarded that year by the foundation. Taken together, the projects represented the most concerted effort yet by the Gates Foundation to overcome the scientific obstacles blocking significant advances against some of the most widespread and deadly health problems afflicting people living in the developing world.[1] Four years into his grant, Flavell was getting tantalizingly close to producing the first laboratory mouse to contain a fully functioning human immune system.
But at the GCGH's annual meeting in 2009, Flavell was told his grant would not be renewed. For more than two decades scientists had been moving in small steps to create a so-called humanized mouse, a laboratory-based surrogate to study human disease in action. Once achieved, Flavell's humanized mouse would give researchers throughout the scientific community a living laboratory model to investigate how the complex human immune system responds to infectious diseases and cancer. It would allow researchers to safely conduct initial tests of new drugs and vaccines against HIV/ AIDS, malaria and tuberculosis among other pernicious ills. Scientists might even be encouraged to try out innovative approaches in the lab rodent that they might not otherwise pursue over concern for risking harm to human test subjects.
"None of the existing animal models adequately addresses the needs of vaccine developers," Flavell and colleagues wrote in a review in 2009.[2] The "lack of suitable animal surrogates creates a seemingly insurmountable barrier to conducting essential preclinical investigations," they wrote.
This argument and the research team's experience in manipulating mouse and human genetics persuaded the GCGH grant reviewers that the project's potential was worth the expensive gamble. Harold Varmus, the Nobel Prize-winning former head of the National Institutes of Health, and chair of the GCGH awards committee, cited the Flavell endeavor as an example of the game-changing research that the Gates Foundation had in mind when it created the GCGH program.
"I would not have even conceived the project if it weren't for the grant from the Grand Challenges," Flavell said in a conversation at his lab in New Haven in 2014.
Despite Flavell's years of producing notable research advances the project was closely monitored, reflecting the Gates Foundation's philosophy of holding grantees accountable for how the award was being spent. As with each of the GCGH grants, the project was required to reach specific milestones in timelines agreed through negotiation between the GCGH staff and each principal investigator like Flavell. The GCGH program required recipients to provide regularly scheduled accountability reports and host site visits by foundation staffers, a type of oversight akin to how the pharmaceutical and biotech industry manages its research projects. "At first we were put off by having the foundation looking so closely over our shoulder," says Elizabeth Eynon, a longtime manager of Flavell's lab. "I now believe it created more pressure and kept us focused."
In addition, each year beginning in 2006, the GCGH grantees were invited to report their progress at a meeting located in a country where the Gates Foundation was courting local research and funding partners. At the 2009 meeting in Arusha, Tanzania, Flavell met with Fil Randazzo, the GCGH project officer assigned to oversee the humanized mouse effort. Randazzo delivered some disturbing news. Since receiving the grant, the Flavell team - using sophisticated genetic engineering research tools - had replaced several genes involved in the mouse immune system with their human counterparts as their first steps to building a complete human immune system functioning in a living laboratory model. Flavell, head of the medical school's immunobiology department and an acclaimed Howard Hughes Medical Investigator, was certain his team had earned another five-year grant.
That was no longer possible, Randazzo told him. After the 2009 financial crisis, funding throughout the Gates Foundation was under pressure. "Our steady and significant projected budget increases disappeared so we had to make some tough prioritization," Randazzo says. "I told Richard that continued funding beyond the original five-year grant was highly unlikely."
"I would not have even conceived the project if it weren't for the grant from the Grand Challenges."
But during the same meeting Flavell was presented an opportunity to revise the goals of the project. During presentations at the gathering Flavell realized that as part of its prioritizing, the Gates Foundation was shifting its support to research most likely to produce treatment advances in the near-term. Flavell's project didn't meet that criteria as its objective was to create multi-purpose animal models other researchers could use in their studies. At the meeting, after several grantees described their efforts to create new vaccines against malaria, Flavell decided to shift his project to create a lab surrogate for malaria researchers.
"So, we were given the opportunity to propose developing a mouse with active human liver and blood cells as well as containing a human immune system," Flavell says. Soon afterwards he contacted one of his collaborators, the biotechnology company Regeneron Inc. Together they agreed to introduce into their mouse the human gene erythropoietin (EPO), a growth factor that stimulates red blood cell production. In 2010, after formally proposing the new direction, Flavell and his team were awarded a three-year $5.7 million extension of their grant to create a chimeric mouse with a human immune system, red blood cells and liver cells. (Chimera was a fire-breathing creature in Greek mythology that was composed of parts of more than one animal.) When the extension expired, the Gates Foundation provided an additional $2.5 million to fund the project through 2015.
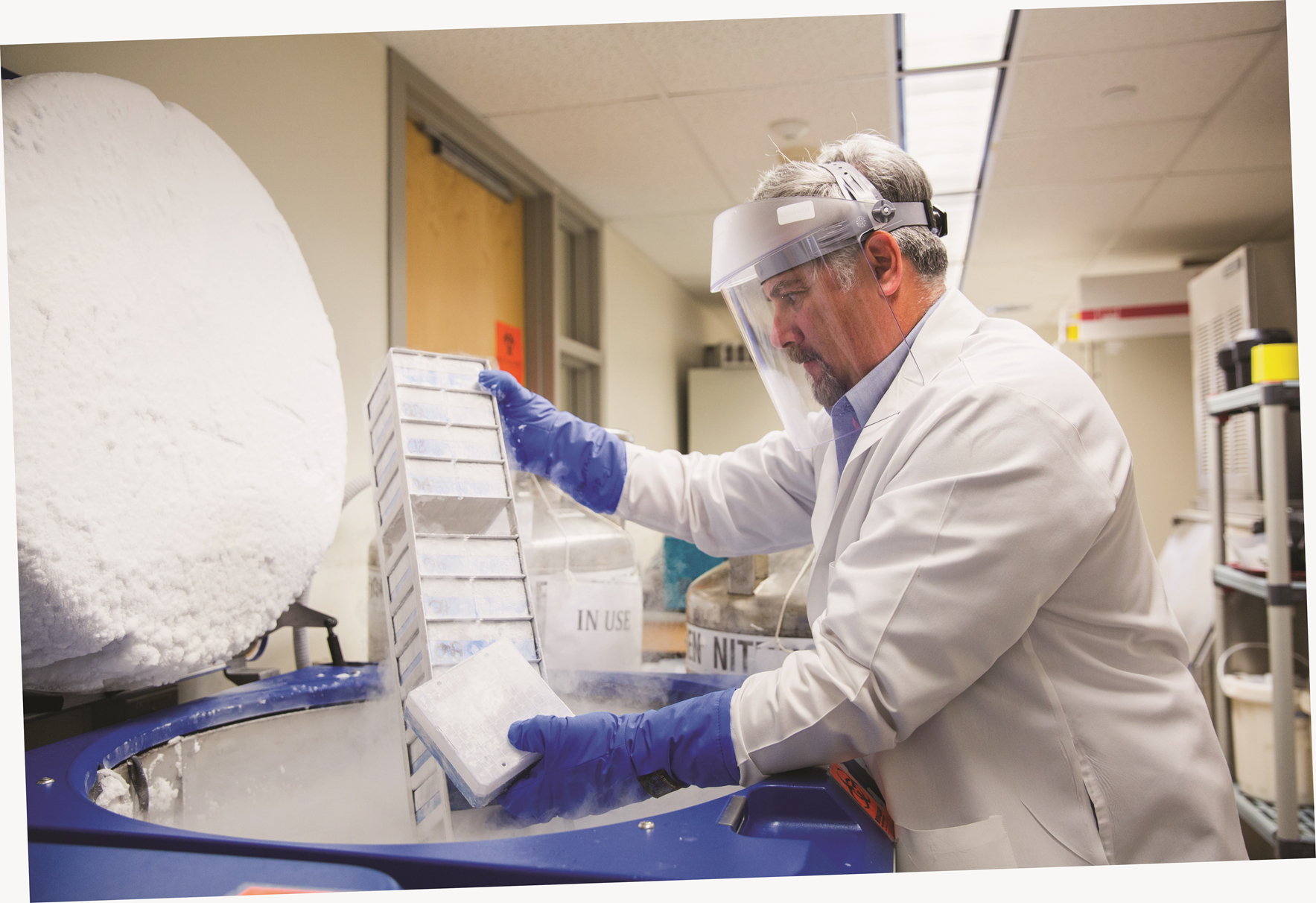
The original objective of the Flavell grant - the explicit reason for creating a humanized mouse - was to produce a rodent model for safely testing new vaccines composed of living viruses or other pathogens. Vaccines based on living pathogens that have been weakened or "attenuated" can stimulate especially potent and long-lasting immunity. For years, researchers have wanted to develop vaccines composed of attenuated versions of HIV, Mycobacterium tuberculosis (the cause of TB) and Plasmodium falciparum (the cause of malaria), mostly because other approaches have failed. But such efforts have been thwarted by the possibility, although remote, that once circulating within humans these weakened pathogens might mutate back into their deadly forms. A humanized mouse, it was proposed in the original grant, might provide a safe way to conduct initial tests of attenuated vaccines.
By the early days of the new century, Flavell's laboratory and researchers elsewhere had already developed a powerful technology to investigate aspects of human biology inside mice. They did this by adding, or "knocking-in," human genes into the immune-deficient rodents' DNA. "The Grand Challenges (in Global Health) gave us the resources to try to flesh out some of the new ideas in the field," Flavell says.
Flavell was inspired by experiments published in 2004[3] by the lab of a young researcher, Markus Manz at the Institute for Research in Biomedicine in Bellinzona, Switzerland. Manz and his colleagues recreated the elements of human immunity by inserting hematopoietic stem cells into the livers of newborn immune-deficient mice. Hematopoietic stem cells (HSC) are produced in the bone marrow and give rise to all types of blood cells including those that carry out immunity. Manz extracted stem and progenitor cells from fetal blood derived from umbilical cords conserved by hospital staff and normally discarded after birth. Earlier attempts using similar approaches to reconstitute human immunity in the mice were hampered because several critical cell types failed to mature. Manz advanced the field by placing the cells directly into the liver of newborn mice, as immune cells are derived developmentally from the early liver. From there the cells naturally migrate to the bone marrow and reconstitute the immune system.
While the Manz model contained more of the complex components of the human immune system than earlier efforts, the amount of certain immune cells was still lower than produced by people, and many didn't survive long. Flavell believed his lab had a good chance of addressing the problem by knocking into the Manz mice human genes encoding growth factor proteins known as "cytokines" that naturally promote immune-cell growth. This proposed solution became the basis of the Grand Challenges in Global Health grant originally awarded to Flavell in collaboration with Manz and Regeneron, which had developed an efficient method for knocking-in genes.
The idea was to initially replace four mouse growth factors with their human counterparts, each of which was responsible for increasing the amount of a specific subset of immune cells. The team decided to carry out the effort by replacing one gene at a time that each encoded a different growth factor. Then, if each knock-in gene spurred higher amounts of the desired immune cells, the researchers' plan was to eventually create one mouse strain containing all four human genes. The first gene introduced produced a cytokine called thrombopoietin, or TPO.[4] Numerous experiments at Yale, in Switzerland and at Regeneron, showed that the mice containing the inserted human TPO generated significantly increased amounts of hematopoietic stem cells in the bone marrow as compared to what the Manz lab previously produced in its studies.
Between 2005 and late 2009, the collaboration engrafted, one at a time, each of the three other cytokines: interleukin 3 (IL3), granulocyte-macrophage colony stimulating factor 2 (GM-GSF), and macrophage colony stimulating factor (M-CSF). By 2011, the team had combined all four growth factors into one mouse, generating a strain that produced almost all the varied subsets of human immune cells. Based on new research elsewhere,[5] the researchers also decided to knock-in the human gene for a protein called signal-regulatory protein, or SIRP, that increased the lifespan of the human cells.[6] In a bit of wry humor researchers named the resulting mouse MISTRG, each letter standing for one of the engrafted human genes plus the knockouts of Rag2 and IL2R gamma.[7]
Creating MISTRG had involved thousands of experiments. Even using Regeneron's industrial knock-in technique, called Velocigene, inserting each of the human genes into their correct location within the mouse genome took hundreds of attempts across many months, Flavell says. Each gene engraftment was made possible by several powerful tricks of nature. One is that two complementary, or homologous, pieces of DNA latch onto one another as part of the natural cell replication process. The scientists decided to exploit this characteristic by attaching DNA from the front and back end of the mouse's own growth-factor genes to the front and back of the human version of the gene. The short lengths of mouse DNA act like the engine and caboose of a long train whose freight cars in between contain the full human gene. Using a microscopic-sized needle and an electrical charge, researchers implanted the combined DNA into the nucleus of embryonic stem cells from mice but adapted to survive in vitro (e.g., in the laboratory). In a successful insertion, the train's cargo - the full human gene - replaces the mouse gene. Researchers then implant the stem cell containing the human gene into an early stage of a mouse embryo.
The additional Gates Foundation funding received was to support the creation of a chimeric mouse containing human liver and blood cells within a human immune environment. As part of the new initiative, oversight of the project was transferred to Omar Vandal who was also managing a portfolio of malaria grants for the malaria group. The malaria group was already supporting several laboratories developing chimeric mice with human livers, including the laboratory of Stefan Kappe at Seattle Biomedical Research Institute. Kappe had previously received a $13.9 million Grand Challenges in Global Health grant in 2005 to develop an attenuated whole parasite malaria vaccine designed to prevent the parasite from transitioning to the bloodstream from infected liver cells. His lab, in collaborations with researchers elsewhere, was using a chimeric mouse to test attenuation of a first-generation version of the vaccine.
As the malaria parasite's life cycle in humans primarily involves infection of liver cells called hepatocytes, and subsequently red blood cells, the researchers began developing strategies to produce one chimeric mouse containing both those cell types. Once that was accomplished the researchers planned to infect the mouse with sporozoites, the infectious form of the parasite Plasmodium falciparum that is most common in Africa and transmitted from one individual to another by the Anopheles gambiae mosquito. When a female Anopheles that carries the malaria parasite bites an individual to get blood to nourish her fertilized eggs, the sporozoites enter the human bloodstream. Soon afterwards, the sporozoites are transported to the liver and infect liver cells. Within those cells the parasite multiplies into new and dangerous forms. After about a week, tens of thousands of these transformed parasites explode out of the liver and into the bloodstream where they infect and destroy red blood cells causing anemia and other health problems that can last for months. Untreated with anti-malarial drugs, the infection can be lethal. If the parasite-infected blood cells reach the brain they can produce a life-threatening cerebral hemorrhage that is often the cause of death for young children.
By 2009, Regeneron had used the knock-in gene technology to introduce human erythropoietin, or EPO into mice. Erythropoietin is a cytokine growth factor produced in the liver and kidneys that spurs hematopoietic stem cells to differentiate into red blood cells. By intercrossing mice, the Flavell group combined all the human genes into one new mouse. The team dubbed the new mouse MISTER G, adding the letter E for EPO. In subsequent lab experiments, the team was able to infect circulating red blood cells extracted from the new Mr. G with parasites.
By this time, Kappe and malaria researchers elsewhere were experimenting with a humanized mouse that contained liver cells developed by a lab run by Markus Grompe at Oregon Health & Science University, Portland, Oregon.[8] As with MISTRG, the Grompe mouse called FRGN was an enhanced version of the original Manz model. In the FRGN mouse, the Grompe team deleted a mouse enzyme that led to build up of a metabolite toxic to hepatocytes, resulting in their destruction. The mouse hepatocytes were then replaced with hepatocytes from human liver donors. In 2011 Kappe successfully infected the FRGN mouse with sporozoites that invaded almost all the engrafted human liver cells and resulted in robust parasite growth in the humanized liver.[9] The FRGN experiments show that "it will be relatively straightforward to add human hepatocytes" to MISTRG to produce a chimeric mouse that can mimic the full lifecycle of the parasite's human infection, Kappe says.
The short lengths of mouse DNA act like the engine and caboose of a long train whose freight cars in between contain the full human gene.
Still several further challenges remained. In particular, the scientists eventually want to create a chimeric mouse that can be infected by Plasmodium vivax, a form of the parasite that causes the majority of malaria infections outside Africa. Unlike the falciparum parasite, vivax parasites often lay dormant in liver cells for many months. Research to create a chimeric mouse that can sustain vivax infections will require further modifications mainly because the blood forms of the parasite only grow in immature red blood cells called reticulocytes. Meanwhile the Kappe lab has already successfully shown vivax liver infections in the human liver-chimeric FRGN mice and demonstrated that the parasite's dormant liver forms indeed persist and activate.[10]
"The current humanized mouse models have already revolutionized the way we do research with human malaria parasites but we can currently only study liver infection and to a limited extent red blood cell infection in isolation," Kappe says. "A combined humanized mouse model that can harbor liver stages, allow transition to blood stages and continuously support blood stage infection would constitute a major advance," the Kappe-Grompe team wrote in early 2014 in a review of the full project's progress.[11]
By 2015, the Flavell lab had produced a mouse model with a stable level of red blood cells that "should be suitable for infection with blood stage vivax infections," Flavell says. His laboratory by this time also was well into advanced plans to combine the liver stage into the MISTERG model which, he says, "bodes well for the generation of a mouse which can offer the study and manipulation of the complete malarial infection."
THE SCIENCE:
Developing human hemato-lymphoid system mice to study human diseases and test candidate vaccines and drugs
Mouse models with humanized biological systems have been developed to promote the translation of preclinical findings into the clinic. Human hemato-lymphoid system mice were originally generated by injecting human CD34+ hematopoietic stem and progenitor cells derived from cord blood directly into newborn mouse livers.[3] However innate immune cells, particularly NK cells, monocytes and macrophages, failed to fully mature and function, likely because they were not well supported by the mouse microenvironment.[12] To address this, Flavell and colleagues introduced selected human cytokines into the mice to better support engraftment of these lineages and more fully recapitulate functional human hematopoietic and immune systems.
Using Regeneron’s VelociGene technology, they systematically replaced four cytokine genes in immune deficient Rag2-|-Il2rg-|- mice with their corresponding human counterparts while retaining the original mouse regulatory elements to ensure their expression in the relevant context. The cytokine genes chosen (human macrophage colony stimulating factor [M-CSF], IL-3, GM-CSF and thrombopoietin [TPO]) were not cross-reactive between humans and mice, and were shown to individually support development of human myeloid cells (M-CSF, IL-3, and GM-CSF) and hematopoietic stem cells (TPO) from CD34+ progenitors.[4], [13], [14] The quadruple knock-in was combined with transgenic expression of human signal regulatory protein (SIRP) α to inhibit mouse phagocytosis of engrafted human cells.[6] The resulting so-called MISTRG mice permitted the robust development of innate immune system cells,[7] boosting the study of human pathogenic diseases, as well as enabling more valuable preclinical testing of candidate vaccines and drugs.
REFERENCES:
[1] Varmus H, Klausner R, Zerhouni E, Acharya T, Daar AS, Singer PA. Public Health. Grand Challenges in Global Health. Science 302, 398 (2003).
[2] Legrand N, Ploss A, Balling R, Becker PD, Barsotti C, Brezillon N, Debarry J, de Jong Y, Deng H, Di Santo JP, Eisenbarth S, Eynon E, Flavell RA, Guzman CA, Huntington ND, Kremsdorf D, Manns MP, Manz MG, Mention JJ, Ott M, Rathinam C, Rice CM, Rongvaux A, Stevens S, Spits H, Strick-Marchand H, Takizawa H, van Lent AU, Wang C, Weijer K, Willinger T, Ziegler P. Humanized mice for modeling human infectious disease: challenges, progress, and outlook. Cell Host Microbe 6, 5 (2009).
[3] [a],[b] Traggiai E, Chicha L, Mazzucchelli L, Bronz L, Piffaretti JC, Lanzavecchia A, Manz MG. Development of a human adaptive immune system in cord blood cell-transplanted mice. Science 304, 104 (2004).
[4] [a],[b] Rongvaux A, Willinger T, Takizawa H, Rathinam C, Auerbach W, Murphy AJ, Valenzuela DM, Yancopoulos GD, Eynon EE, Stevens S, Manz MG, Flavell RA. Human thrombopoietin knock-in mice efficiently support human hematopoiesis in vivo. Proc Natl Acad Sci U S A 108, 2378 (2011).
[5] Takenaka K, Prasolava TK, Wang JC, Mortin-Toth SM, Khalouei S, Gan OI, Dick JE, Danska JS. Polymorphism in Sirpa modulates engraftment of human hematopoietic stem cells. Nat lmmunol. 8, 1313 (2007).
[6] [a],[b] Strowig T, Rongvaux A, Rathinam C, Takizawa H, Borsotti C, Philbrick W, Eynon EE, Manz MG, Flavell RA. Transgenic expression of human signal regulatory protein alpha in Rag2-/-gamma(c)-/- mice improves engraftment of human hematopoietic cells in humanized mice. Proc Natl Acad Sci U S A 108, 13218 (2011).
[7] [a],[b] Rongvaux A, Willinger T, Martinek J, Strowig T, Gearty SV, Teichmann LL, Saito Y, Marches F, Halene S, Palucka AK, Manz MG, Flavell RA. Development and function of human innate immune cells in a humanized mouse model. Nat Biotechnol. 32, 364 (2014).
[8] Azuma H, Paulk N, Ranade A, Dorrell C, Al-Dhalimy M, Ellis E, Strom S, Kay MA, Finegold M, Grompe M. Robust expansion of human hepatocytes in Fah-/-/Rag2-/-/Il2rg-/- mice. Nat Biotechnol. 25, 903 (2007).
[9] Vaughan AM, Mikolajczak SA, Wilson EM, Grompe M, Kaushansky A, Camargo N, Bial J, Ploss A, Kappe SH. Complete Plasmodium falciparum liver-stage development in liver-chimeric mice. J Clin Invest. 122, 3618 (2012).
[10] Mikolajczak SA, Vaughan AM, Kangwanrangsan N, Roobsoong W, Fishbaugher M, Yimamnuaychok N, Rezakhani N, Lakshmanan V, Singh N, Kaushansky A, Camargo N, Baldwin M, Lindner SE, Adams JH, Sattabongkot J, Kappe SH. Plasmodium vivax liver stage development and hypnozoite persistence in human liver-chimeric mice. Cell Host Microbe 17, 526 (2015).
[11] Kaushansky A, Mikolajczak SA, Vignali M, Kappe SH. Of men in mice: the success and promise of humanized mouse models for human malaria parasite infections. Cell Microbial. 16, 602 (2014).
[12] Rongvaux A, Takizawa H, Strowig T, Willinger T, Eynon EE, Flavell RA, Manz MG. Human hemato-lymphoid system mice: current use and future potential for medicine. Annu Rev Immunol. 31, 635 (2013).
[13] Rathinam C, Poueymirou WT, Rojas J, Murphy AJ, Valenzuela DM, Yancopoulos GD, Rongvaux A, Eynon EE, Manz MG, Flavell RA. Efficient differentiation and function of human macrophages in humanized CSF-1 mice. Blood 118, 3119 (2011).
[14] Willinger T, Rongvaux A, Takizawa H, Yancopoulos GD, Valenzuela DM, Murphy AJ, Auerbach W, Eynon EE, Stevens S, Manz MG, Flavell RA. Human IL-3/GM-CSF knock-in mice support human alveolar macrophage development and human immune responses in the lung. Proc Natl Acad Sci U S A 108, 2390 (2011).