HiSeq Machines [DNA sequencers) at the Wellcome Trust Sanger Institute.
LEARNING FROM THE HUMAN GENOME HOW PROTECTIVE IMMUNITY AGAINST MALARIA WORKS
- Jan 1, 2016
- Download Retrospective
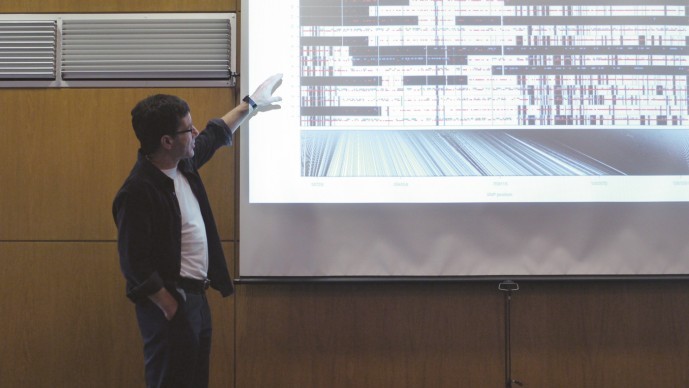
Dominic Kwiatkowski in 2013 presenting at the annual Genomic Epidemiology of Malaria meeting. Photo courtesy of Victoria Cornelius
Dominic Kwiatkowski, Oxford University/Sanger Institute, United Kingdom
Malaria kills almost 600,000 people a year, most of whom are African children, according to the World Health Organization. Yet, millions of other people recover from an infected mosquito bite without succumbing to the life-threatening complications, especially with access to effective drug regimens. But scientists also have wondered if certain individuals respond especially well to treatment or even get well without drugs because they harbor a natural immunity. If components of that natural defense can be identified, scientists have speculated, they may provide clues to better medicines or even a potent preventive vaccine.
"The central role of genetics in immunity to malaria has long been recognized," says Dominic Kwiatkowski, head of the malaria program at the Wellcome Trust Sanger Institute and a professor at the University of Oxford. "But previously we lacked the scientific tools to investigate this in any detail."
Over a decade ago, Kwiatkowski set out to identify the genetic factors that determine protective immunity against malaria. His idea was to use new DNA analysis technologies to sift through the genomes of thousands of people infected with malaria, looking for small stretches of DNA sequences that predict whether an individual will die or whether they will survive. The hope was to discover the genes that are involved in innate protection. ''At the time it was considered a radical idea," Kwiatkowski says.
Equally radical was the way that Kwiatkowski proposed to obtain the many thousands of blood samples required for major discoveries: forming a collaborative network of researchers in malaria-endemic countries to collect and share clinical and genetic data. "When we started out, the research community was fragmented, with different groups pursuing small studies on their own samples," says Kwiatkowski. "What was needed, and what we wanted to do is integrate data from multiple populations and geographical locations, and standardize ways of collecting and analyzing the data."
This strategy garnered $8 million funding from the Bill & Melinda Gates Foundation's Grand Challenges in Global Health program, complemented with a matching amount from the UK's Wellcome Trust. Kwiatkowski's first move was to create a global research consortium by funding research groups in 20 countries and then providing them with the expertise to conduct their own clinical and genetic studies. He named the data-sharing partnership the Malaria Genomic Epidemiology Network, or MalariaGEN.[1]
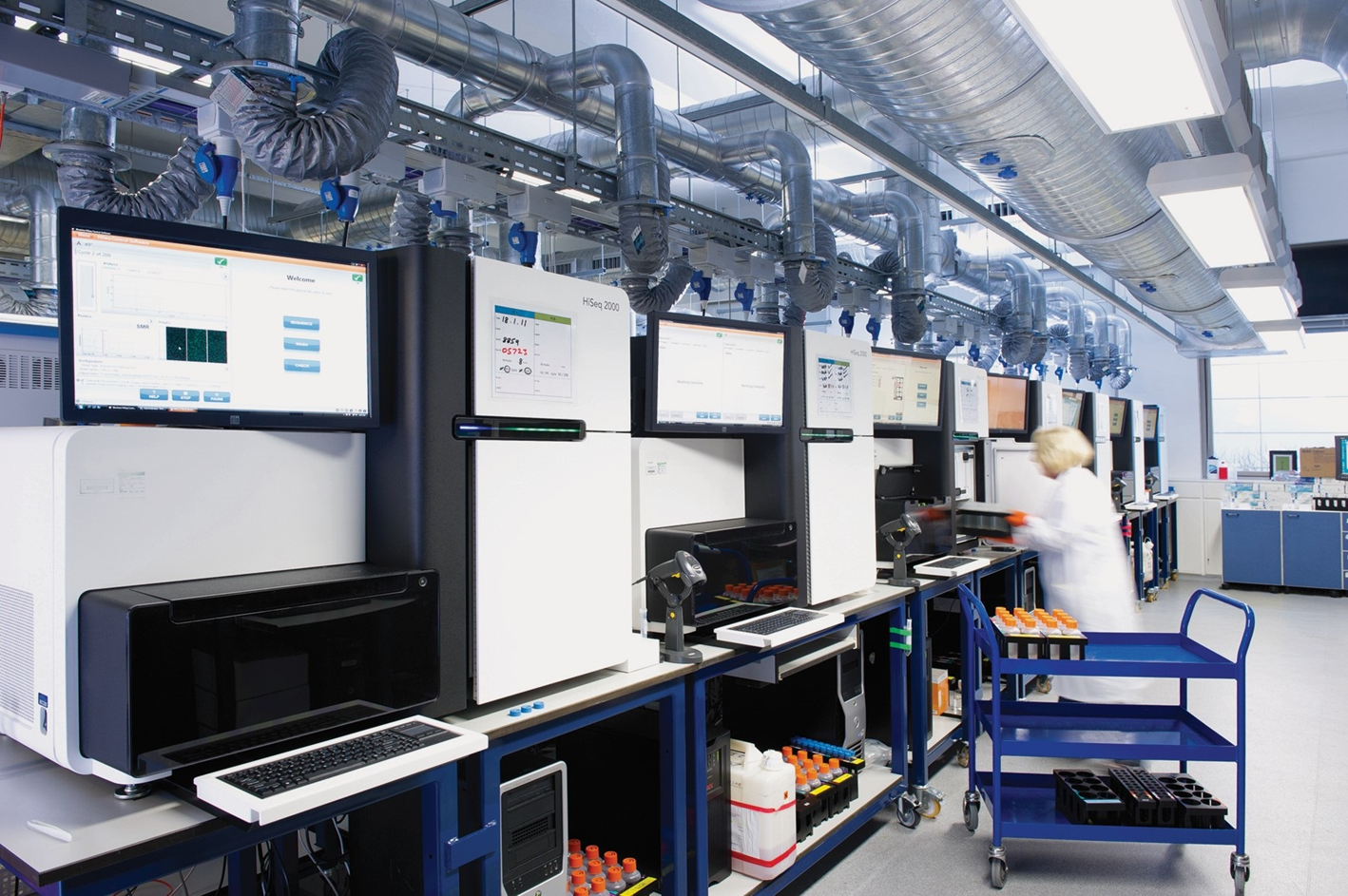
Eight years down the road, with data from over a hundred thousand samples collected around the world, evidence of the biology underlying innate immunity is starting to emerge. In early June 2013, Kwiatkowski gathered 50 scientists from across MalariaGEN's far-flung network at the campus of the Wellcome Trust's Sanger Institute outside Cambridge, UK. For three days he led a review of the gigantic set of clinical and genetic data that had been generated. The starting point was an analysis of over 20 genetic variants that had previously been reported in the literature to be associated with resistance to malaria. Some of these variants were confirmed in the MalariaGEN sample set comprising 12,000 cases of severe malaria and 17,000 controls from 12 different populations in Africa and Asia. But others were not, and these may have been experimental artifacts arising from the small sample size of previous studies.[2] "When we started MalariaGEN, there were many who doubted whether genetic associations could be believed," says Kwiatkowski. "Large multicenter studies allowed us for the first time to sort out the wheat from the chaff, and to have confidence that we are observing true biological effects. That is a huge step forward for the field."
The meeting's high point came when attendees were told of the latest new discovery, which revealed the strongest evidence so far that the clinical outcome of a malaria infection depends on the specific route that the parasite uses to get itself into human red blood cells, called erythrocytes. Understanding exactly how Plasmodium falciparum parasites invade erythrocytes remains one of the great mysteries of malaria research and is a holy grail for many vaccine developers. It is believed that this can happen via several pathways, each initiated by a lock and key mechanism whereby the parasite displays a binding protein that is recognized by a receptor protein on the surface of the erythrocyte. The first such erythrocyte receptor exploited by the malaria parasite was discovered over thirty years ago to be a sugar-coated protein called glycophorin A, and it was later found that other forms of glycophorin can also act as receptors for invasion. The MalariaGEN investigators have discovered that the risk of an African child developing severe malaria is strongly predicted by variations in the region of the human genome that contains the genes for three different forms of glycophorin, including glycophorin A. "That's an important find," says Christopher Plowe, a key malaria researcher at the University of Maryland.
In conversations over lunch, tea breaks, and drinks at the Institute's conference center, Kwiatkowski emphasized the mammoth effort it entailed. He chose his words carefully, speaking in the confident, measured manner of a scientist comfortable with taking on big risks. He made clear that the glycophorin discovery, despite its scientific value, is just one step towards his ultimate goal. "It's a novel observation in understanding the complex interactions that occur between humans and the parasite," Kwiatkowski tells me. "Still, we're at the very beginning of what we need to do."
Kwiatkowski's plan, as stated in his grant proposal back in 2004, was to harness the fast-advancing DNA sequencing technology to identify the many different human genes that act together to determine whether the outcome of a malaria infection is death or survival. He reasoned that this type of clinical research in human populations who are naturally exposed to infection would provide vital clues for vaccine developers that could not be obtained by experimental studies in the laboratory. "The glycophorin discovery is a great example," he says, "because it looks like we now have an entirely new way of getting at the question of how parasites invade erythrocytes, and how this may vary from one individual to another."
But Kwiatkowski concedes that researchers will need to uncover much more about the biological interactions between parasite and human to be useful for vaccine developers. Many malaria researchers agree. "I’m a huge fan of the Grand Challenges, but it was unrealistic to expect a vaccine to emerge after only five or eight years," says Plowe. One of the main technical challenges that MalariaGEN has had to deal with is the great genetic diversity of populations affected by malaria, particularly in Africa,[3],[4],[5] which makes it much more difficult to discover genetic associations than is the case for studies of commondiseases in European populations.
"The central role of genetics in immunity to malaria has long been recognized,” says Dominic Kwiatkowski. "But previously we lacked the scientific tools to investigate this in any detail.”
By 2008, as it became clear that the search for the genetic underpinning of natural immunity was going to be even more arduous than expected, Kwiatkowski concedes to some "despondency and despair," he says. Urged to press on despite these challenges, most notably by Michael Gottlieb, the grant's avuncular project officer at the Foundation for the National Institutes of Health, Kwiatkowski decided to increase the statistical power of the study by analyzing the largest possible number of samples that funds would allow. In his original proposal for Grand Challenges funding, he budgeted for analysis of 1000 individuals at 100,000 variable positions (known as SNPs) in the human genome. By the end of the grant, he and his team had analyzed 20,000 individuals at 2.5 million SNPs, a 500-fold increase in the scale of data compared to what was originally proposed. This was achieved largely by cutting costs and additional funding from the Wellcome Trust and the UK Medical Research Council, as well as an additional $2 million from the Grand Challenges in Global Health program.
Although the Grand Challenges grant has come to the end, Kwiatkowski says that the MalariaGEN network is now working on another 20 regions of the genome that show differences between children who get severely ill and those who don't. Funding for the additional work will mostly come from the Wellcome Trust, which is also supporting studies of genome variation in the parasite and the mosquito to complement the human genetic studies. This is based on the pioneering work of Kwiatkowski's team, published in 2012 in Nature,[6] which analyzed P. falciparum diversity in natural infections by genome sequencing of human blood samples. Although this important finding wasn't funded by the Grand Challenges grant, it resulted in large part from the genetics information and resources developed by MalariaGEN. In addition, MalariaGEN coordinates P. falciparum 3000 Genomes Consortium (Pf3k) and the Anopheles gambiae 1000 Genomes Consortium (Ag1000G), which enable groups around the world to get genome sequence data on the parasite and mosquito samples that they are collecting for their own research, while making the aggregated data openly available through user-friendly web applications.[7]
An important practical application of the parasite-sequencing work was published by Kwiatkowski's team and their MalariaGEN partners in Nature Genetics in 2013. By comparing sequencing data from almost a thousand parasite samples from different locations in Southeast Asia and West Africa, they discovered several distinct populations of parasites resistant to the most powerful malaria drug, artemisinin, that have recently emerged in Western Cambodia.[8] "Artemisinin is the mainstay of malaria control and if resistance spreads to Africa it will be a global health disaster," says Olivo Miotto, a member of Kwiatkowski's team who is based in Bangkok, and the first author of the Nature Genetics study.
"We have disrupted the old approaches and brought big data and genomics into malaria research,” says Kwiatkowski. "That's no small achievement.”
Although the project's full value will take years to measure, there is little to doubt that MalariaGEN's most enduring products are the people now in Africa and Asia with the skills to carry out sophisticated genetics research. MalariaGEN's work on capacity building has resulted in local leadership of this field of research, notably through the African Plasmodium Diversity Network (PDNA), a group of African researchers who are driving forward genetic studies of drug resistance across the continent and have recently been awarded $7 million funding to establish an African-led training scheme as part of the Wellcome Trust DELTAS initiative.
Eight researchers in malaria-endemic countries have PhD projects supported by MalariaGEN data fellowships. There is no better example than Rajika Dewasurendra, a 34-year-old PhD candidate in molecular biology at the University of Colombo in Sri Lanka, who spent seven years in the country's Kataragama farming district, dodging elephants while slogging through jungle pathways to reach distant villages speckled among rice-paddy fields. Rajika initially was a MalariaGEN "data fellow" recruited to collect blood samples from villagers who had survived a malaria outbreak in 1998 when 40 to 50 patients were diagnosed each day with the disease.
Her assignment was to track down 1,500 people who didn't perish at the time to help MalariaGEN determine if the makeup of their immune system protected them. Rajika has far surpassed her assignment, devising a series of experiments that may identify better ways to treat patients.
At first, "our biggest challenge was to find the exact people" in a database of survivors in order to get blood samples, Rajika says. Traveling among the farm villages, she soon learned that some people's whereabouts weren't known, others had passed away, moved, or worked in the fields during the day, only returning home well after dark. Despite this, she obtained 1,200 samples.
As it was too far to transfer research material to the university lab in Colombo, her group installed a centrifuge, freezer and refrigerator to separate serum and store samples. Because of the difficulty in keeping the samples under freezing temperatures during the six-hour trip to Colombo, Rajika set up her own small laboratory to extract and analyze DNA herself, skills she gained by attending annual MalariaGEN workshops held outside Sri Lanka.
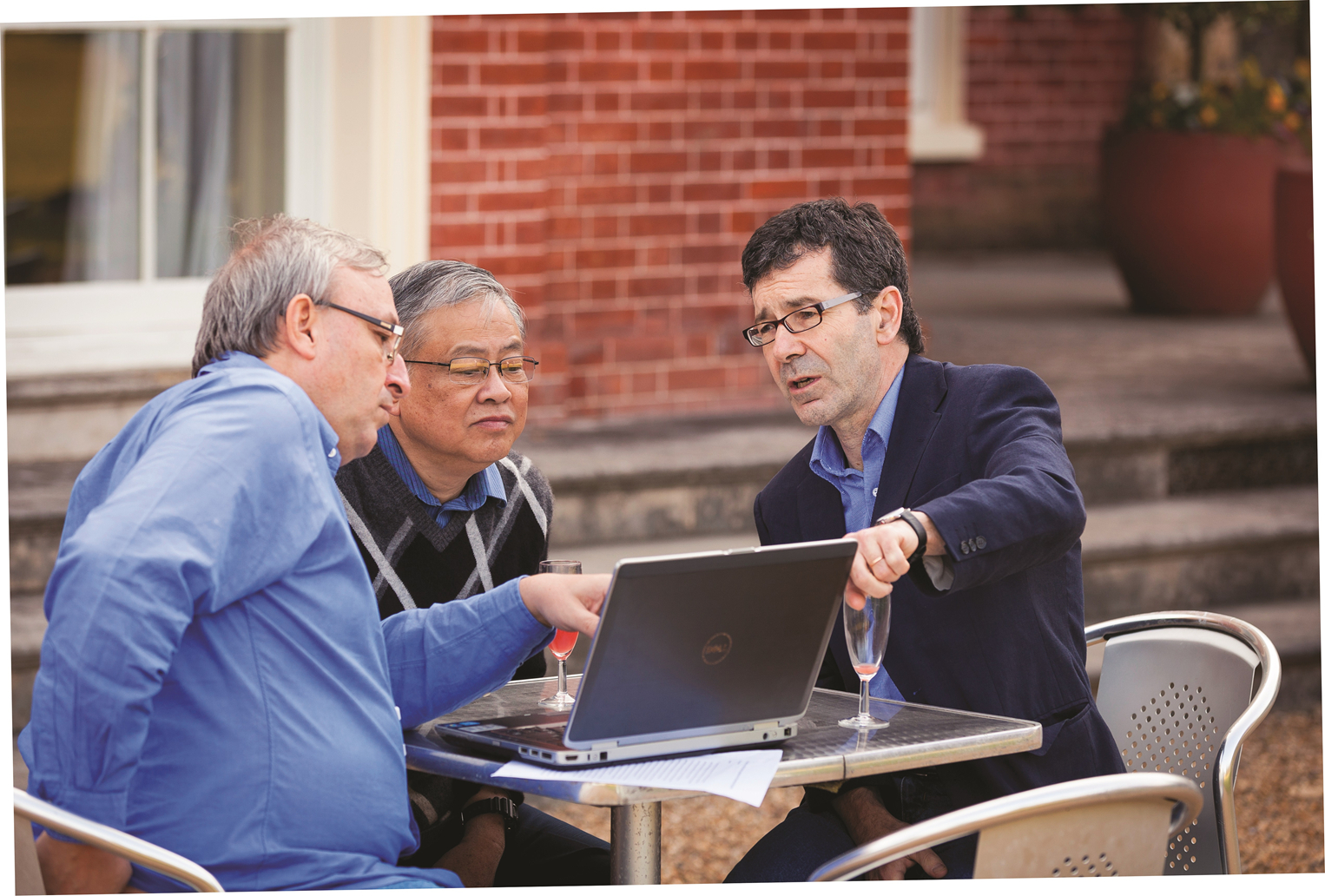
Dominic Kwiatkowski discusses results with his collaborators Olivo Miotto (left) and Tran Tinh Hien (middle).
During this time, she embarked on her doctoral studies supported by the Sri Lanka government and university grants. In research that was recently published, the one-time data fellow identified an enzyme deficiency among people especially vulnerable to malaria.[9] The finding might someday lead to a blood test to identify patients who don't fare well with routine treatments, says Nadira Karunaweera, Rajika's mentor and MalariaGEN's principal investigator at the university.
"We've watched Rajika blossom during the past eight years into an independent scientist," says Karunaweera. "She'll be a resource here for decades to come."
"We have disrupted the old approaches and brought big data and genomics into malaria research," says Kwiatkowski. "That's no small achievement. The fact that people are sharing data in this way is in itself a paradigm shift."
THE SCIENCE:
Analyzing genomic variation in Plasmodium falciparum to study artemisinin resistance
Within only ten years, the malaria parasite Plasmodium falciparum has evolved resistance to the frontline anti-malarial drug artemisinin. This resistance is currently restricted to Southeast Asia.[10] To identify the genetic factors involved in artemisinin resistance, Kwiatkowski and colleagues developed large-scale sequencing tools[6] and utilized material generated by the collaborative research network set-up through funding from the GCGH program.[1] They analyzed genome variation in parasites from 825 malaria cases around the principal site of artemisinin resistance in western Cambodia, and found at least three distinct subpopulations of resistant parasites.Western Cambodia.[8]
A major step forward came when others subsequently identified the propeller domain of the kelch13 gene as a marker of artemisinin resistance.[11] And then more recently, allelic replacement experiments showed that kelch13 mutations actually confer artemisinin resistance.[12] Transcriptome analysis of 1043 clinical P. falciparum isolates suggested an underlying mechanism whereby artemisinin resistant parasites exhibit changes in cellular stress responses that result in delayed onset of cell death following drug exposure.[13]
An unexpected diversity of over twenty different kelch13 mutations has been associated with artemisinin resistance in Southeast Asia, all appearing to have emerged independently.[10] Kwiatkowski's team and partners performed the largest genome-wide association study of artemisinin resistance based on analysis of 1,612 samples from 15 locations in Cambodia, Vietnam, Laos, Thailand, Myanmar and Bangladesh.[14] They discovered that resistance-causing mutations are most likely to occur in parasites with nonsynonymous polymorphisms in fd (ferredoxin), arps10 (apicoplast ribosomal protein S10), mdr2 (multidrug resistance protein 2) and crt (chloroquine resistance transporter).
This finding and others help explain the defined geographic distribution of artemisinin resistance and provide markers for surveillance of the spread of parasites with this predisposing genetic background.[14],[15]
REFERENCES:
[1] [a],[b] Malaria Genomic Epidemiology Network. A global network for investigating the genomic epidemiology of malaria. Nature 456, 732 (2008).
[2] Malaria Genomic Epidemiology Network; Malaria Genomic Epidemiology Network. Reappraisal of known malaria resistance loci in a large multicenter study. Nat Genet. 46, 1197 (2014).
[3] Jallow M, Teo YY, Small KS, Rockett KA, Deloukas P, Clark TG, Kivinen K, Bojang KA, Conway DJ, Pinder M, Sirugo G, Sisay-Joof F., Usen S, Auburn S, Bumpstead SJ, Campino S, Coffey A, Dunham A, Fry AE, Green A, Gwilliam R, Hunt SE, Inouye M, Jeffreys AE, Mendy A, Palotie A, Potter S, Ragoussis J, Rogers J, Rowlands K, Somaskantharajah E, Whittaker P, Widden C, Donnelly P, Howie B, Marchini J, Morris A, SanJoaquin M, Achidi EA, Agbenyega T, Allen A, Amodu O, Corran P, Djimde A, Dolo A, Doumbo OK, Drakeley C, Dunstan S, Evans J, Farrar J, Fernando D, Hien TT, Horstmann RD, Ibrahim M, Karunaweera N, Kokwaro G, Koram KA, Lemnge M, Makani J, Marsh K, Michon P, Modiano D, Molyneux ME, Mueller I, Parker M, Peshu N, Plowe CV, Puijalon O, Reeder J, Reyburn H, Riley EM, Sakuntabhai A, Singhasivanon P, Sirima S, Tall A, Taylor TE, Thera M, Troye-Blomberg M, Williams TN, Wilson M, Kwiatkowski DP; Wellcome Trust Case Control Consortium; Malaria Genomic Epidemiology Network. Genome-wide and fine-resolution association analysis of malaria in West Africa. Nat Genet. 41 ,657 (2009).
[4] Teo YY, Small KS, Kwiatkowski DP. Methodological challenges of genome-wide association analysis in Africa. Nat Rev Genet. 11, 149 (2010).
[5] Band G, Le QS, Jostins L, Pirinen M, Kivinen K, Jallow M, Sisay-Joof F, Bojang K, Pinder M, Sirugo G, Conway DJ, Nyirongo V, Kachala D, Molyneux M, Taylor T, Ndila C, Peshu N, Marsh K, Williams TN, Alcock D, Andrews R, Edkins S, Gray E, Hubbart C, Jeffreys A, Rowlands K, Schuldt K, Clark TG, Small KS, Teo YY, Kwiatkowski DP, Rockett KA, Barrett JC, Spencer CC; Malaria Genomic Epidemiology Network. Imputation-based meta-analysis of severe malaria in three African populations. PLoS Genet. 9, e1003509 (2013).
[6] [a],[b] Manske M, Miotto O, Campino S, Auburn S, Almagro-Garcia J, Maslen G, O'Brien J, Djimde A, Doumbo O, Zongo I, Ouedraogo JB, Michon P, Mueller I, Siba P, Nzila A, Borrmann S, Kiara SM, Marsh K, Jiang H, Su XZ, Amaratunga C, Fairhurst R, Socheat D, Nosten F, Imwong M, White NJ, Sanders M, Anastasi E, Alcock D, Drury E, Oyola S, Quail MA, Turner DJ, Ruano-Rubio V, Jyothi D, Amenga-Etego L, Hubbart C, Jeffreys A, Rowlands K, Sutherland C, Roper C, Mangano V, Modiano D, Tan JC, Ferdig MT, Amambua-Ngwa A, Conway DJ, Takala-Harrison S, Plowe CV, Rayner JC, Rockett KA, Clark TG, Newbold CI, Berriman M, MacInnis B, Kwiatkowski DP. Analysis of Plasmodium falciparum diversity in natural infections by deep sequencing. Nature. 487, 375 (2012).
[8] [a],[b] Miotto O, Almagro-Garcia J, Manske M, MacInnis B, Campino S, Rockett KA, Amaratunga C, Lim P, Suon S, Sreng S, Anderson JM, Duong S, Nguon C, Chuor CM, Saunders D, Se Y, Lon C, Fukuda MM, Amenga-Etego L, Hodgson AV, Asoala V, Imwong M, Takala-Harrison S, Nosten F, Su XZ, Ringwald P, Ariey F, Dolecek C, Hien TT, Boni MF, Thai CQ, Amambua-Ngwa A, Conway DJ, Djimde AA, Doumbo OK, Zongo I, Ouedraogo JB, Alcock D, Drury E, Auburn S, Koch O, Sanders M, Hubbart C, Maslen G, Ruano-Rubio V, Jyothi D, Miles A, O'Brien J, Gamble C, Oyola SO, Rayner JC, Newbold CI, Berriman M, Spencer CC, McVean G, Day NP, White NJ, Bethell D, Dondorp AM, Plowe CV, Fairhurst RM, Kwiatkowski DP. Multiple populations of artemisinin-resistant Plasmodium falciparum in Cambodia. Nat Genet. 45,648 (2013).
[9] Dewasurendra RL, Rockett KA, Fernando SD, Carter R, Kwiatkowski DP, Karunaweera ND; MalariaGEN Consortium. G6PD gene variants and its association with malaria in a Sri Lankan population. Malar J. 14 (2015).
[10] [a],[b] Ashley EA, Dhorda M, Fairhurst RM et al., Tracking Resistance to Artemisinin Collaboration (TRAC). Spread of artemisinin resistance in Plasmodium falciparum malaria. N Engl J Med. 371, 411 (2014).
[11] Ariey F, Witkowski B, Amaratunga C, Beghain J, Langlois AC, Khim N, Kim S, Duru V, Bouchier C, Ma L, Lim P, Leang R, Duong S, Sreng S, Suon S, Chuor CM, Bout DM, Ménard S, Rogers WO, Genton B, Fandeur T, Miotto O, Ringwald P, Le Bras J, Berry A, Barale JC, Fairhurst RM, Benoit-Vical F, Mercereau-Puijalon O, Menard D. A molecular marker of artemisinin-resistant Plasmodium falciparum malaria. Nature 505, 50 (2014).
[12] Straimer J, Gnädig NF, Witkowski B, Amaratunga C, Duru V, Ramadani AP, Dacheux M, Khim N, Zhang L, Lam S, Gregory PD, Urnov FD, Mercereau-Puijalon O, Benoit-Vical F, Fairhurst RM, Ménard D, Fidock DA. Drug resistance. K13-propeller mutations confer artemisinin resistance in Plasmodium falciparum clinical isolates. Science 347 ,428 (2015).
[13] Mok S, Ashley EA, Ferreira PE, Zhu L, Lin Z, Yeo T, Chotivanich K, Imwong M, Pukrittayakamee S, Dhorda M, Nguon C, Lim P, Amaratunga C, Suon S, Hien TT, Htut Y, Faiz MA, Onyamboko MA, Mayxay M, Newton PN, Tripura R, Woodrow CJ, Miotto O, Kwiatkowski DP, Nosten F, Day NP, Freiser PR, White NJ, Dondorp AM, Fairhurst RM, Bozdech Z. Drug resistance. Population transcriptomics of human malaria parasites reveals the mechanism of artemisinin resistance. Science 347, 431 (2015).
[14] [a],[b] Miotto O, Amato R, Ashley EA, Macinnis B, Almagro-Garcia J, Amaratunga C, Lim P, Mead D, Oyola SO, Dhorda M, Imwong M, Woodrow C, Manske M, Stalker J, Drury E, Campino S, Amenga-Etego L, Thanh TN, Tran HT, Ringwald P, Bethell D, Nosten F, Phyo AP, Pukrittayakamee S, Chotivanich K, Chuor CM, Nguon C, Suon S, Sreng S, Newton PN, Mayxay M, Khanthavong M, Hongvanthong B, Htut Y, Han KT, Kyaw MP, Faiz MA, Fanello CI, Onyamboko M, Mokuolu OA, Jacob CG, Takala-Harrison S, Plowe CV, Day NP, Dondorp AM, Spencer CC, McVean G, Fairhurst RM, White NJ, Kwiatkowski DP. Genetic architecture of artemisinin-resistant Plasmodium falciparum. Nat Genet. 47, 226 (2015).
[15] Kwiatkowski D. Malaria genomics: tracking a diverse and evolving parasite population. Int Health 7, 82 (2015).